Research
Functions of N-glycan branches
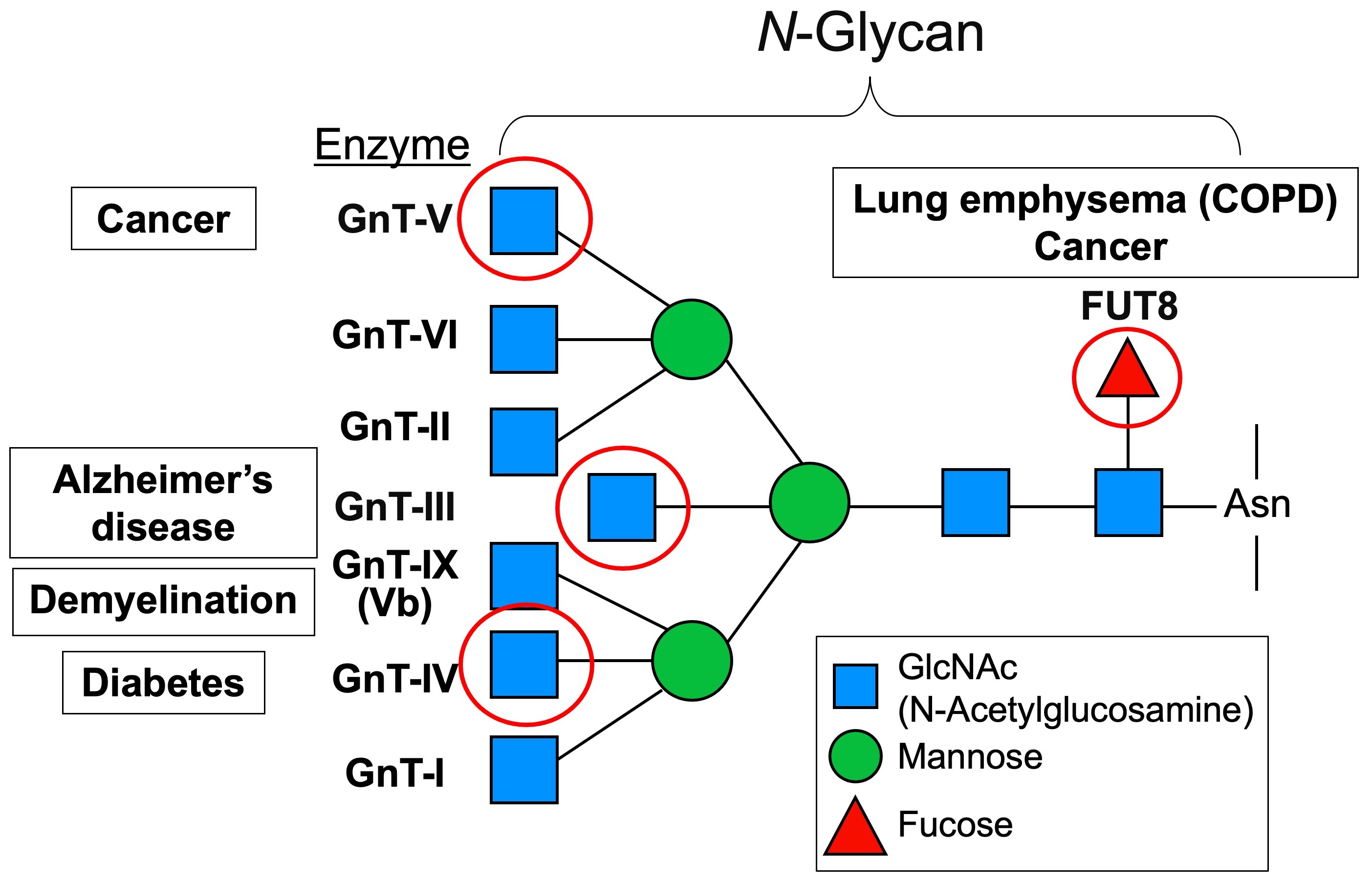
Fig. 1 Branched structures of N-glycans, their biosynthetic enzymes and implications in disease.
We work on expression, functions, and disease-involvement of N-glycans attached to proteins. Particularly, we focus on branched structures of glycans.
N-glycosylation is fundamental protein modifications with large structural variations (Fig. 1). Moreover, formation of each branch is protein-selective and occurs on limited proteins. However, it is poorly understood at molecular level how each branch functions and how such protein selectivity is accomplished.
We tackle these questions by focusing on biosynthetic branching enzymes (glycosyltransferases). We expect that our results will finally lead to development of new therapeutics against glycan-related diseases.
Bisecting GlcNAc, an accelerator of Alzheimer’s disease
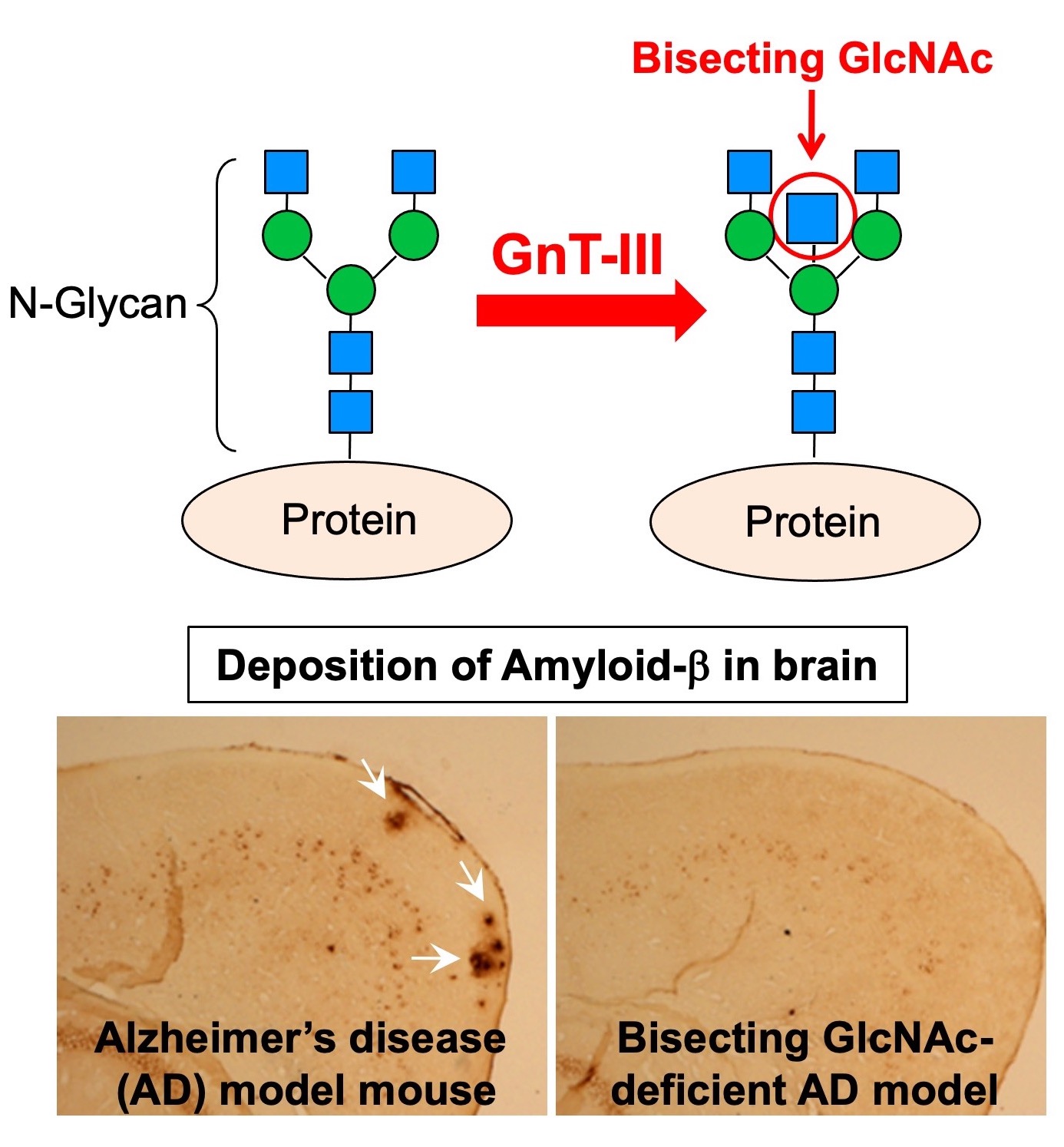
Fig. 2. (Upper) Structure and biosynthetic enzyme of bisecting GlcNAc. (Lower) Deposition of amyloid-β (Aβ) in brain of an AD model mouse. The Aβ level is reduced in bisecting GlcNAc-deficient mice.
(Reference:Kizuka et al., EMBO Mol. Med., 2015)
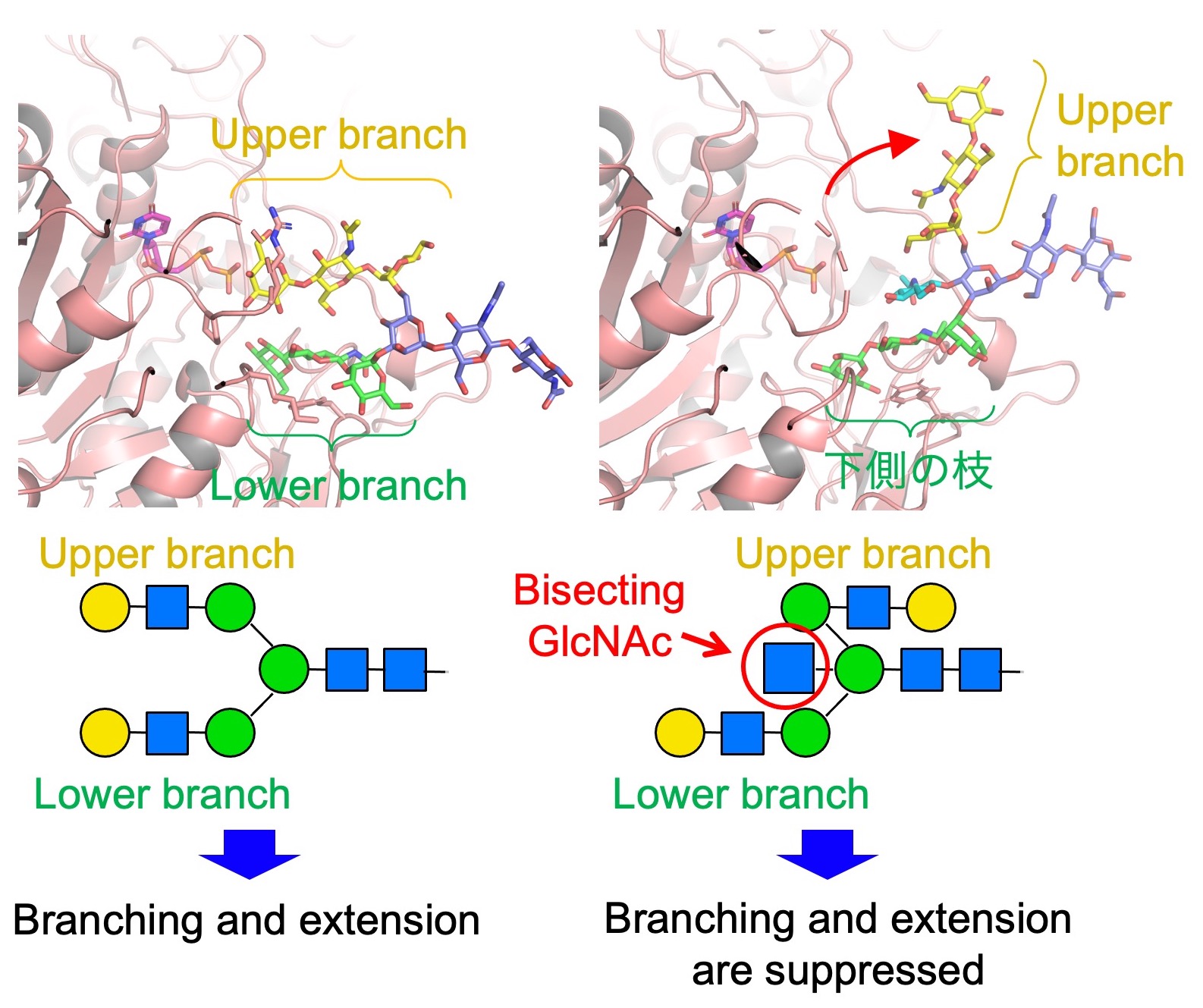
Fig. 3. 3D structure of the upper branch of N-glycan is changed by the presence of bisecting GlcNAc. This suppresses further extension and branching of N-glycans.
(Reference:Nakano et al., Mol. Cell Proteomics, 2019)
Bisecting GlcNAc is one of N-glycan branches (Fig. 2, upper). We have revealed that this glycan structure is profoundly involved in development and progression of Alzheimer’s disease (AD). AD is known to be caused by deposition of Amyloid-β (Aβ) peptide in brain, and we found that bisecting GlcNAc-deficient mice have reduced Ab deposition and improved AD pathology (Fig. 2, lower). As a mechanism, we found that Aβ-producing enzyme BACE1 is modified with bisecting GlcNAc and that BACE1 function is positively regulated by this glycan modification. Furthermore, the levels of bisecting GlcNAc are increased in AD patients, suggesting its involvement in AD progression in human. However, we still do not know how BACE1 function is regulated by bisecting GlcNAc at a molecular level. We now examine this mechanism and search for inhibitor of the biosynthetic enzyme of bisecting GlcNAc, GnT-III.
We also examine the physiological functions of bisecting GlcNAc. By looking at 3D structure of bisecting GlcNAc-containing glycans (Fig. 3, upper), we found that the upper branch in N-glycan is pointed to the opposite direction by the presence of bisecting GlcNAc, compared with other N-glycans without bisecting GlcNAc (Fig. 3, lower). This results in suppression of further extension and branching of N-glycans which could usually occur in non-bisected glycans. Therefore, bisecting GlcNAc has a suppressive role for N-glycan biosynthesis.
We now investigate the functions of bisecting GlcNAc in neurons and kidney where bisecting GlcNAc is highly expressed and also examine the regulation mechanisms of GnT-III functions.
β1,6 branch, an accelerator of cancer
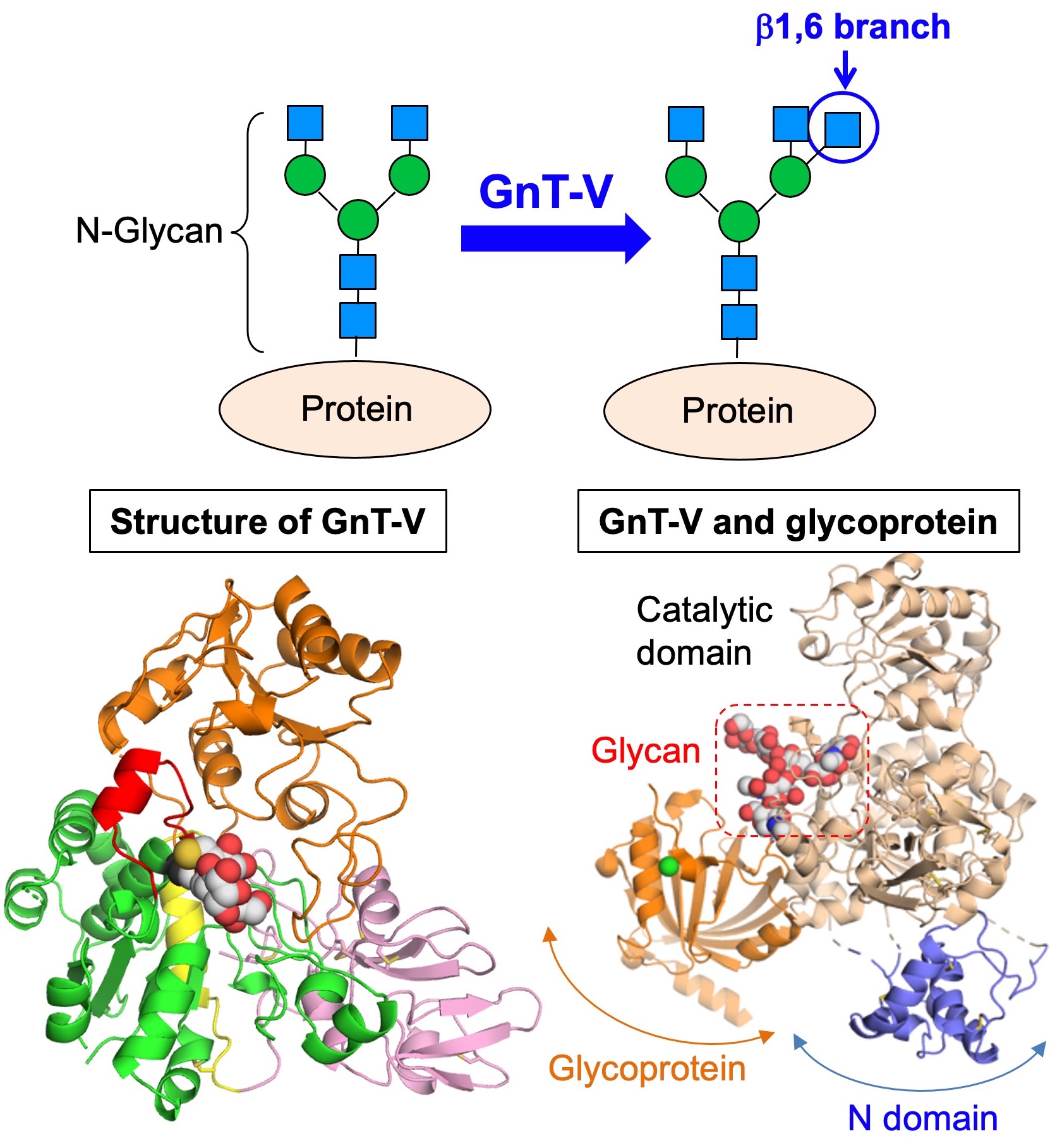
Fig. 4. (Upper) Structure and biosynthetic enzyme of β1,6 branch. (Lower left) Crystal structure of GnT-V. (Lower right) Docking model of GnT-V and a glycoprotein substrate.
(Reference:Nagae et al., Nat. Commun., 2018)
(Reference:Osuka et al., J. Biol. Chem., 2022)
We also focus on other branches in N-glycan which are highly involved in cancer. Particularly, β1,6-branch (Fig. 4, upper) synthesized by GnT-V enzyme is known to promote cancer growth and metastasis. Therefore, it has been considered as a promising drug target for a long time.
We for the first time revealed the 3D crystal structures of GnT-V in collaboration with an expert of structural biology (Dr. Masamichi Nagae) (Fig. 4, lower left). This provided us with basic information regarding how GnT-V recognizes substrate glycans and biosynthesizes β1,6-branch. Furthermore, the clarification of the structure will allow us to design specific inhibitors of GnT-V. We Indeed try to develop GnT-V inhibitors in collaboration with organic chemists.
However, the detailed mechanisms are still poorly understood how GnT-V selects target glycoproteins and works in living cells. To solve this question, we recently found that N domain in GnT-V (Fig. 4, lower right) is important. Mutant GnT-V lacking N domain almost lacks activity toward glycoproteins while it almost fully retains activity toward glycans. This suggests that GnT-V recognizes its substrate proteins through N domain.
Now we try to clarify the mechanism of glycoprotein recognition by GnT-V as well as the regulation mechanisms of GnT-V functions.
Core fucose, a versatile sugar branch
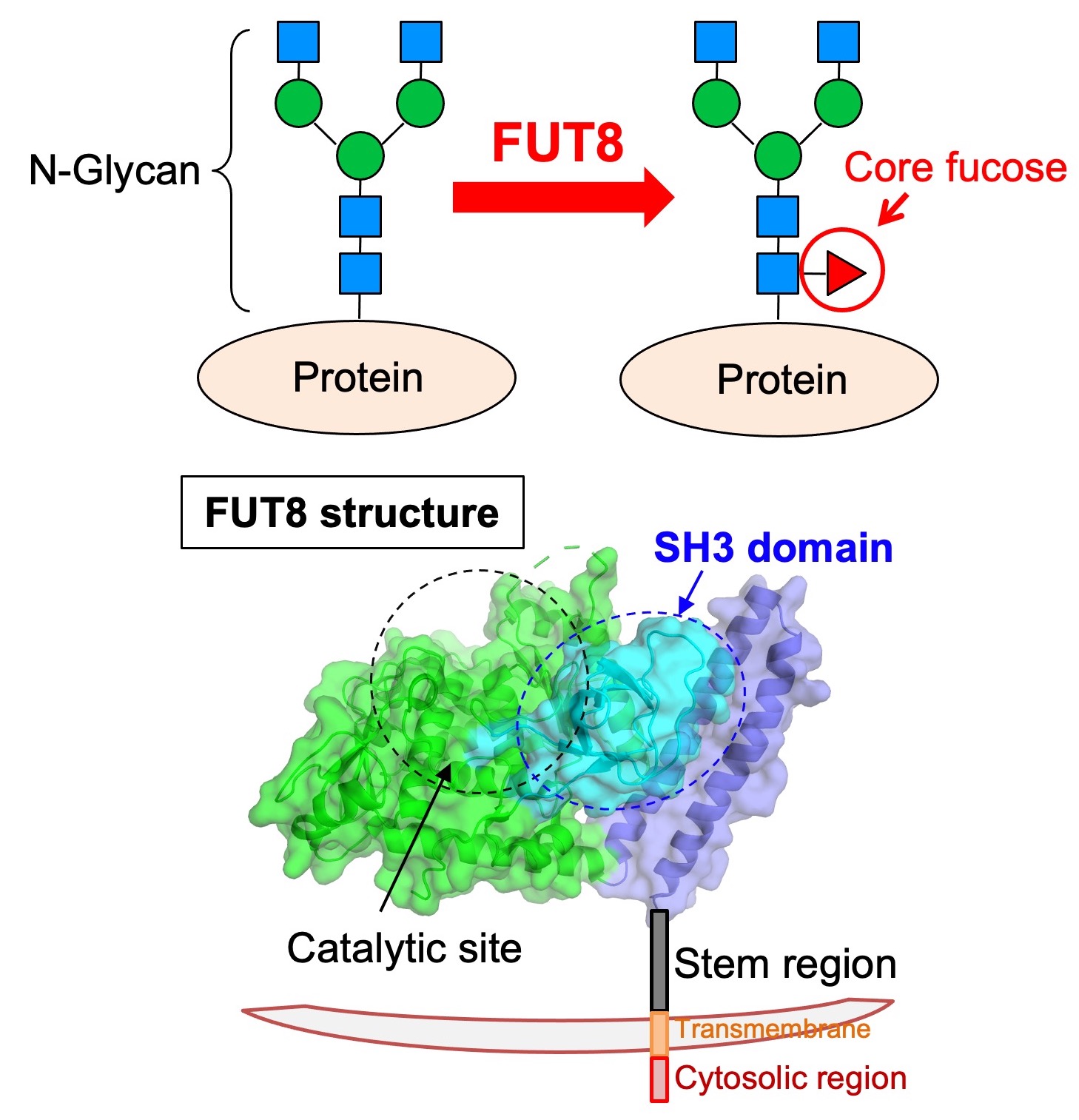
Fig. 5. (Upper) Structure and biosynthetic enzyme of core fucose. (Lower) Crystal structure of FUT8. FUT8 has unique SH3 domain and stem region other than catalytic domain.
(Reference:Tomida et al., J. Biol. Chem., 2020)
We also work on “core fucose” that is a fucose branch on the core part of N-glycan. Core fucose is biosynthesized by an enzyme called FUT8 (Fig. 5, upper), and is known to be involved in chronic obstructive pulmonary disease (COPD), as FUT8-deficient mice show emphysema phenotype. In addition, core fucose was reported to promote lung cancer and melanoma. Furthermore, a core fucose-targeted technique is clinically used in which anti-tumor activity of antibody drug is drastically elevated by removing core fucose from antibody drug. Therefore, core fucose is highly related to diagnosis and therapy of these diseases.
On the other hand, the regulation mechanisms of FUT8 functions remain to be clarified. We examine the mechanisms of how FUT8 functions are regulated in cells, by focusing on 3D structures and regulatory proteins. FUT8 3D structure is already known, and it has unique SH3 domain near the catalytic domain (Fig. 5, lower). We recently found that the SH3 domain is necessary for the enzyme activity of FUT8 and interaction with RPN1 protein. Now we investigate the binding mode and significance of this FUT8-RPN1 interaction.
β1,4 branch related to diabetes
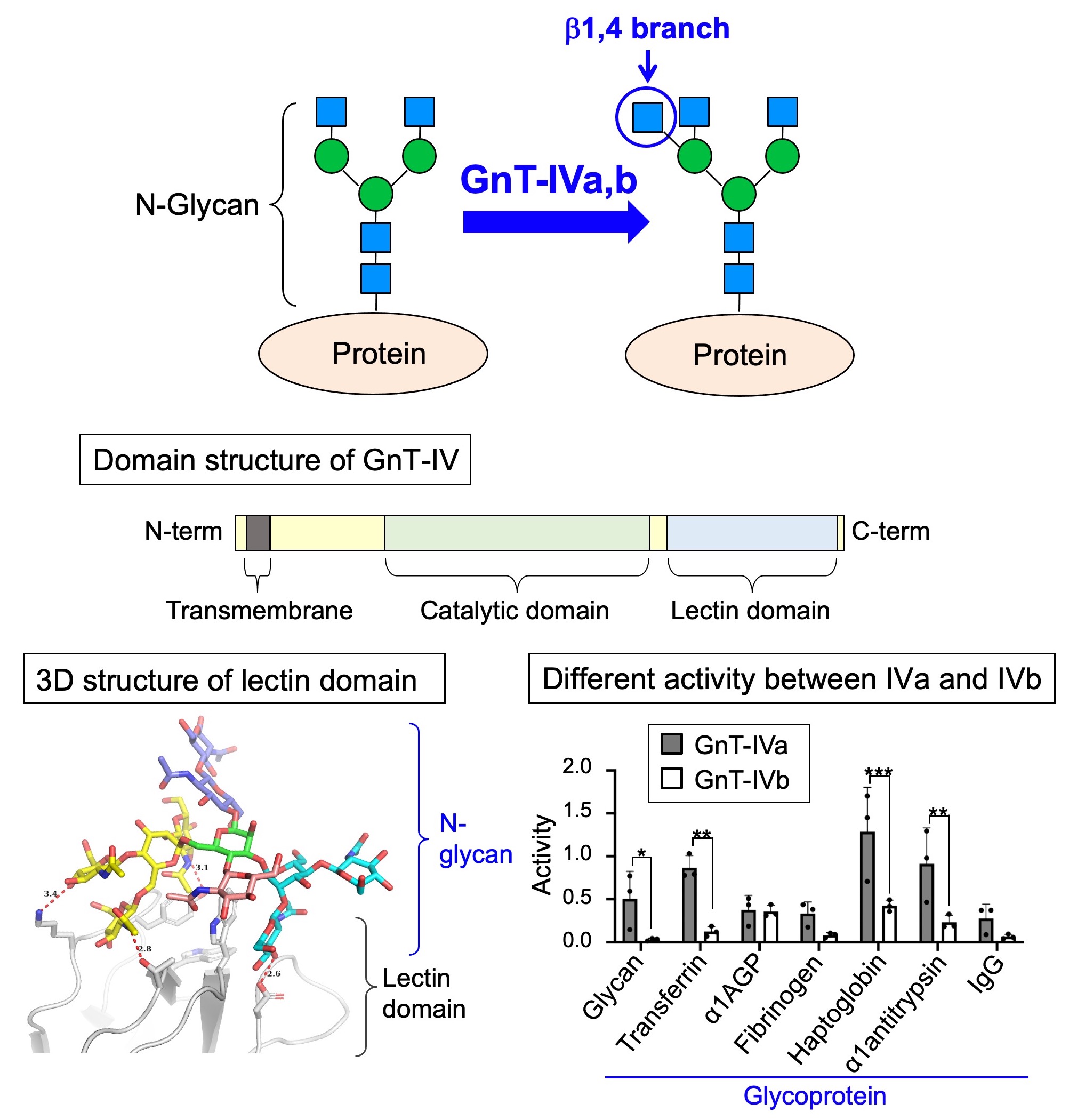
Fig. 6. (Upper) Structure and biosynthetic enzyme of β1,4 branch. (Middle) Domain structure of GnT-IV. (Lower left) 3D structure of GnT-IV lectin domain. (Lower right) Activity of GnT-IVa and –IVb toward various glycoproteins.
(Reference:Nagae et al., Commun. Biol., 2022)
(Reference:Osada et al., J. Biol. Chem., 2022)
Another topic is β1,4 branch, that is related to diabetes. This branch structure is biosynthesized by either one of two similar enzymes, GnT-IVa or GnT-IVb (Fig. 6, upper). As GnT-IVa-deficient mice show impairments of pancreatic functions, leading to diabetic phenotypes with high blood glucose levels, this branch is expected to be one of drug targets for diabetes. On the other hand, it remains largely unclear how GnT-IVa and IVb synthesize glycans in cells and how functions of two similar enzymes, GnT-IVa and IVb, are different.
We recently found that GnT-IV has a unique lectin domain which is not present in other related glycosyltransferases (Fig. 6, middle). Moreover, the lectin domain was found to bind with specific glycans (Fig. 6, lower left), which is required for enzyme activity of the catalytic domain. Since such lectin domain has not been found in other glycosyltranserases involved in N-glycan synthesis, this suggests that GnT-IV has a new mode of action. In addition, we established a new assay system for measuring activity toward glycoproteins, and found that GnT-IVa and IVb have substantially different preferences for glycoprotein substrates (Fig. 6, lower right). This indicates that each of these two similar enzymes has intrinsic functions.
Other disease- and brain-related branches
In addition, we also work on other branches which are involved in diseases and brain functions. Particularly, a branch produced by GnT-IX is studied (Fig. 1). We mainly focus on the molecular mechanisms of regulation of their activity and functions, in collaboration with Japanese and international collaborators.
New glycan probe
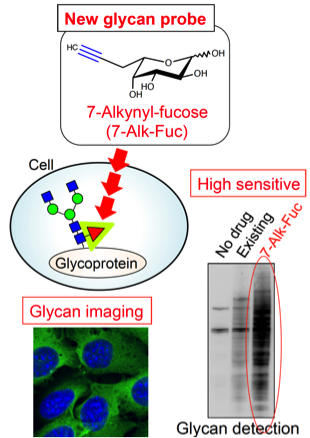
Fig. 7. Highly sensitive detection of glycans with a new fucose analog
(Reference:Kizuka et al., Cell Chem. Biol., 2016)
It is essential for a glycobiology study to detect a glycan of interest. Although common detection methods use antibodies or lectins, we focus on a different method using a combination of sugar analog and click chemistry. This method will enable us to easily detect specific glycans. But because of relatively recent discovery, the method remains to be improved in terms of sensitivity and specificity. So far, we have developed a novel fucose analog which can detect fucosylated glycans with higher sensitivity that are involved in cancer and COPD (Fig. 7). Moreover, we try to develop other new glycan probes. We aim at developing new drug targets and disease biomarkers by using these new probes.
New inhibitor of glycan function
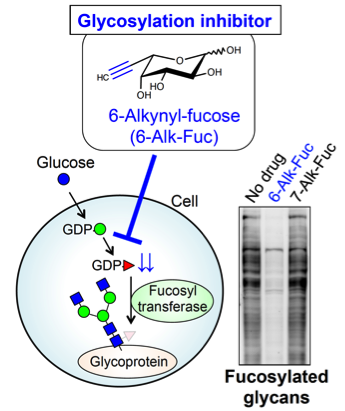
Fig. 8. A new fucosylation inhibitor
(Reference:Kizuka et al., Cell Chem. Biol., 2017)
Even if we could reveal that a certain glycan is involved in disease, we could not cure the disease without a way to exogenously control that process. In addition, that control method will also be required to clarify the function or disease involvement of a specific glycan. Thus, compounds are useful which can inhibit or enhance the glycan functions. We develop inhibitory compounds for glycan functions, especially by focusing on sugar analogs. So far, we have discovered a novel fucose analog which can selectively inhibit the biosynthesis of fucosylated glycans and revealed its action mechanism (Fig. 8). We also showed that this compound suppressed invasive property of cancer cells. Furthermore, in collaboration with Dr. Hidenori Tanaka in Gifu Univ., we developed sugar nucleotide analogs as candidate inhibitors of GnT-V (Vibhute et al., BBA Gen. Subj., 2022). These results suggest that development of novel glycosylation inhibitor will contribute to both basic science and therapeutic application.
Other research projects
GPI (Glycosyl Phosphatidyl Inositol) anchor
We work on biosynthesis and functions of GPI, a unique glycolipid attached to proteins, particularly focusing on prion and neurological diseases (reference: Hirata et al., J. Biol. Chem., 2022). We also work on intracellular trafficking of GPI anchored proteins.
Small extracellular vesicle (sEV) and glycan
Collaborative work with Kenichi Suzuki Lab (iGCORE) on expression and functions of glycans in sEVs, including exosomes. sEVs draw attentions of many researchers, as they function for cell-cell communications.
Arginine methylation and glycan
Collaborative work with Assistant Prof. Misuzu Hashimoto (Faculty of Applied Biol. Sci., Gifu Univ.). We work on relationships between glycans/glycoproteins and arginine methylation which is also known as a posttranslational modification of proteins (reference: Hashimoto et al., BBA Gen. Subj., 2020).
Biosynthetic regulation of HNK-1 (Human Natural Killer-1) glycan
We study on regulation mechanisms of HNK-1 glycan that is specifically expressed in brain and required for learning and memory functions. We have found that GnT-III suppresses HNK-1 biosynthesis in tissue-dependent manners (reference: Kawade et al., Molecules, 2021).